Surface Organometallic Chemistry
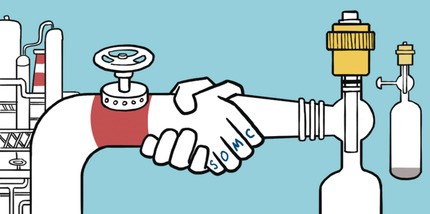
Heterogeneous catalysts are usually prepared via the dispersion of a metal salt/precursor onto a support, followed by heating under a gas stream. Such catalysts contain a broad distribution of metal coordination environments, of which often only a small fraction is active in catalysis, thereby making characterization at the molecular difficult and preventing rational catalyst development. Homogeneous catalysts on the other hand have well-defined active sites, leading to higher selectivities while enabling more rational catalyst development through structure-activity relationships.
Surface Organometallic Chemistry (SOMC) is a powerful tool used in our group to generate well-defined surface sites, thereby bridging the gap between homogeneous and heterogeneous catalysts. This approach treats the surface of the catalyst support as a ligand and aims at controlling its reactivity towards tailored molecular precursor complexes. A key aspect of SOMC is based on controlling the density and the coordination environment of metal sites through grafting of a tailored molecular precursor onto the surface OH groups of an oxide support. The initial OH density is usually controlled via a thermal treatment at high temperatures, and the subsequent grafting step is typically carried out in a solvent at lower temperature, usually at room temperature (Figure 1a). When combined with thermolytic molecular precursors (TMPs) that can lose their organic ligands quite readily upon thermal treatment, SOMC provides access to supported isolated metal sites with defined oxidation state and nuclearity inherited from the precursor (Figure 1b). Imposed by the surface, these sites bear an unusual coordination environment strongly resembling that of the active sites of supported metal oxides. However, they typically contain a higher proportion of active sites making structure-activity relationship possible. These supported isolated sites thus constitute ideal models for supported metal oxides – an important class of industrial catalysts used in olefin metathesis (triolefin processs), olefin epoxidation (Shell process), ethylene polymerization (Phillips catalysts) and propane dehydrogenation (Catofin and related processes). Such SOMC/TMP-derived model systems can provide detailed information about the structure of active sites in industrial catalysts, their mode of initiation and deactivation, as well as the role of the support and specific thermal treatment on the final activity of the catalyst [1]. Using this methodology, we have prepared well-defined silica-supported tungsten imido olefin metathesis catalysts and developed detailed structure-activity-relationships [2, 3]. Furthermore, we have prepared well-defined silica-supported tungsten(VI) oxo surface sites as model systems for WO3/SiO2 for olefin metathesis and managed to propose an activation mechanism [4] Using an SOMC/TMP approach, we have also prepared silica-supported dinuclear Cr(III) sites as a model system for the Phillips catalyst (CrO3 dispersed on silica) and demonstrated that ethylene polymerization involves an initial heterolytic activation of ethylene on Cr-O bonds [5]
Furthermore, SOMC/TMP can be used to generate and investigate supported metal nanoparticles. Grafting of a TMP with a reducible metal center followed by treatment under hydrogen leads to small and narrowly dispersed monometallic nanoparticles (Figure 1c). Alternatively, starting from well-defined isolated sites containing also adjacent surface OH groups, grafting of a second metal and treatment under hydrogen allows us to prepare either supported monometallic particles with tailored interfaces (Figure 1d) or alloyed nanoparticles (Figure 1e). Therefore, SOMC allows us to generate tailored catalytic materials with molecular strategies in which questions of sizes, interfaces and compositions can be addressed [6, 7] This approach allowed us to demonstrate that the nature and the Lewis acidity of sites at the surface of metal oxides and doped metal oxides in Cu-based catalysts for the conversion of CO2 to methanol have profound effects on the reaction outcome [8] In addition, we prepared a silica-supported alloyed Pd-Ga catalyst with high activity and selectivity and were able to show that this is due to a dynamic redox process of Ga (de-/re-alloying) which promotes the stability of reaction intermediates in CO2-to-methanol hydrogenation [9].
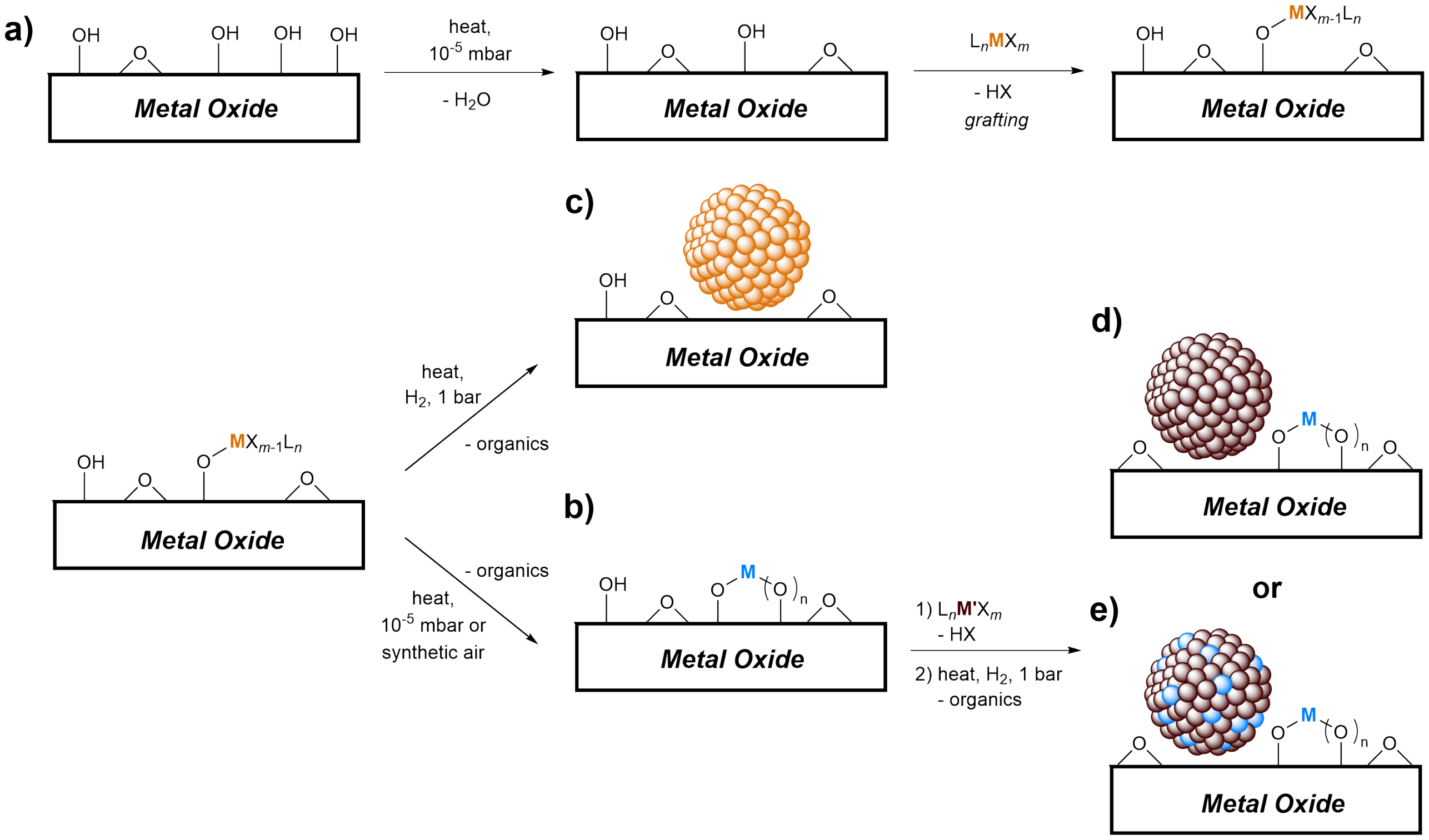
Selected Literature
(1) Copéret, C.; Comas-Vives, A.; Conley, M. P.; Estes, D. P.; Fedorov, A.; Mougel, V.; Nagae, H.; Núñez-Zarur, F.; Zhizhko, P. A. Surface Organometallic and Coordination Chemistry toward Single-Site Heterogeneous Catalysts: Strategies, Methods, Structures, and Activities. Chemical Reviews 2016, 116 (2), 323-421. DOI: 10.1021/acs.chemrev.5b00373.
(2) Mougel, V.; Santiago, C. B.; Zhizhko, P. A.; Bess, E. N.; Varga, J.; Frater, G.; Sigman, M. S.; Copéret, C. Quantitatively Analyzing Metathesis Catalyst Activity and Structural Features in Silica-Supported Tungsten Imido–Alkylidene Complexes. Journal of the American Chemical Society 2015, 137 (20), 6699-6704. DOI: 10.1021/jacs.5b03344.
(3) Pucino, M.; Mougel, V.; Schowner, R.; Fedorov, A.; Buchmeiser, M. R.; Copéret, C. Cationic Silica-Supported N-Heterocyclic Carbene Tungsten Oxo Alkylidene Sites: Highly Active and Stable Catalysts for Olefin Metathesis. Angewandte Chemie International Edition 2016, 55 (13), 4300-4302. DOI: https://doi.org/10.1002/anie.201510678 (acccessed 2024/07/12).
(4) Mougel, V.; Chan, K.-W.; Siddiqi, G.; Kawakita, K.; Nagae, H.; Tsurugi, H.; Mashima, K.; Safonova, O.; Copéret, C. Low Temperature Activation of Supported Metathesis Catalysts by Organosilicon Reducing Agents. ACS Central Science 2016, 2 (8), 569-576. DOI: 10.1021/acscentsci.6b00176.
(5) Conley, M. P.; Delley, M. F.; Siddiqi, G.; Lapadula, G.; Norsic, S.; Monteil, V.; Safonova, O. V.; Copéret, C. Polymerization of Ethylene by Silica-Supported Dinuclear CrIII Sites through an Initiation Step Involving C-H Bond Activation. Angewandte Chemie International Edition 2014, 53 (7), 1872-1876. DOI: external page https://doi.org/10.1002/anie.201308983 (acccessed 2024/07/12).
(6) Copéret, C. Single-Sites and Nanoparticles at Tailored Interfaces Prepared via Surface Organometallic Chemistry from Thermolytic Molecular Precursors. Accounts of Chemical Research 2019, 52 (6), 1697-1708. DOI: 10.1021/acs.accounts.9b00138.
(7) Docherty, S. R.; Copéret, C. Deciphering Metal–Oxide and Metal–Metal Interplay via Surface Organometallic Chemistry: A Case Study with CO2 Hydrogenation to Methanol. Journal of the American Chemical Society 2021, 143 (18), 6767-6780. DOI: 10.1021/jacs.1c02555.
(8) Noh, G.; Lam, E.; Bregante, D. T.; Meyet, J.; Šot, P.; Flaherty, D. W.; Copéret, C. Lewis Acid Strength of Interfacial Metal Sites Drives CH3OH Selectivity and Formation Rates on Cu-Based CO2 Hydrogenation Catalysts. Angewandte Chemie International Edition 2021, 60 (17), 9650-9659. DOI: https://doi.org/10.1002/anie.202100672.
(9) Docherty, S. R.; Phongprueksathat, N.; Lam, E.; Noh, G.; Safonova, O. V.; Urakawa, A.; Copéret, C. Silica-Supported PdGa Nanoparticles: Metal Synergy for Highly Active and Selective CO2-to-CH3OH Hydrogenation. JACS Au 2021, 1 (4), 450-458. DOI: 10.1021/jacsau.1c00021.