Dynamic Nuclear Polarization
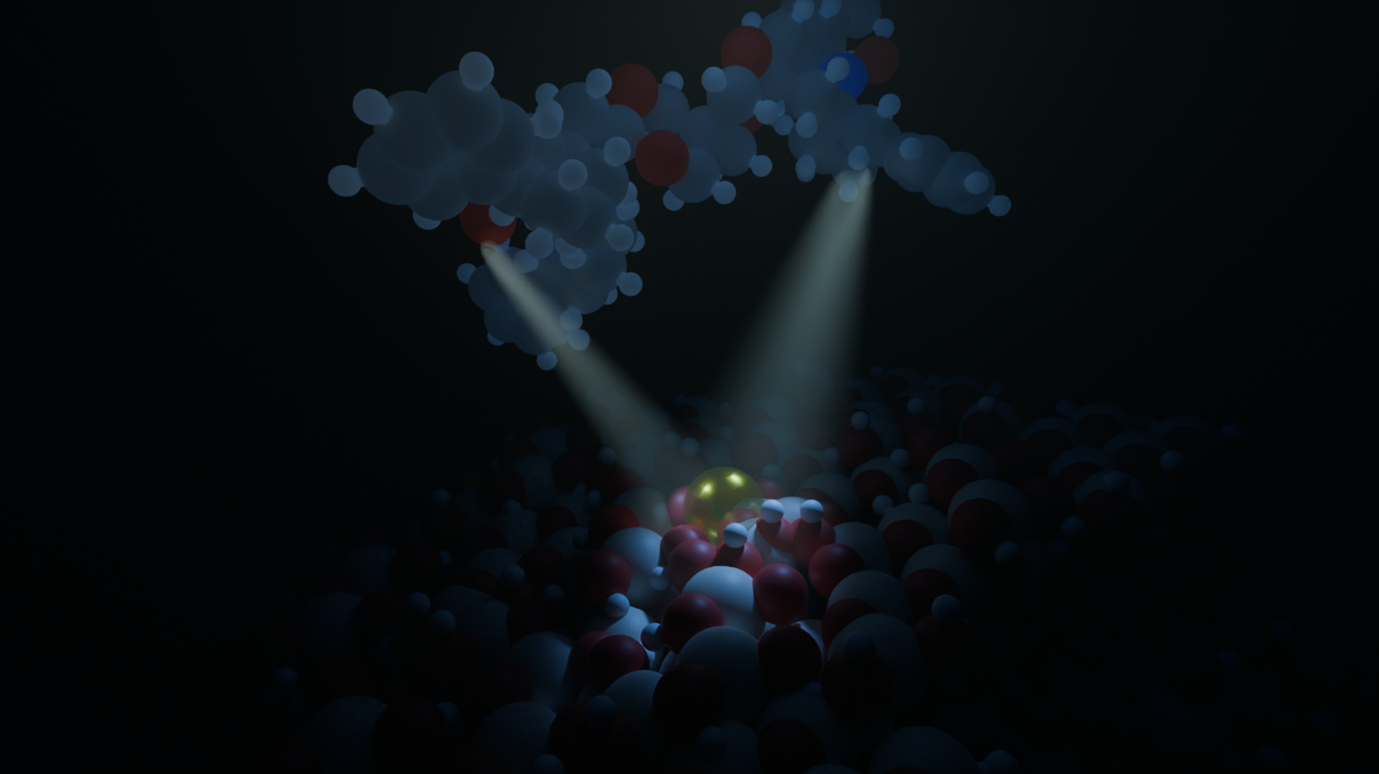
Nuclear magnetic resonance (NMR) spectroscopy is a powerful tool for understanding the structure of compounds and materials, but is often limited by its intrinsic low sensitivity. This issue is exacerbated when studying surface sites that only constitute a (low) fraction of a sample (few %). In that context, dynamic nuclear polarization (DNP) enhances the sensitivity of NMR spectroscopy by transferring the intrinsically high polarization of paramagnetic electron spins to neighboring nuclear spins, in particular protons. Enhancements up to two orders of magnitude (theoretical maximum 660 that corresponds to g¬e/gH) can be achieved at 100 K. In 2010, our group in collaboration with Emsley, Lesage et al. introduced the concept of DNP-surface enhanced NMR spectroscopy (DNP-SENS), where a radical dispersed in a frozen glassy matrix and adsorbed on a material via incipient wetness impregnation, was shown to selectively enhance the NMR-signal of surface species.[1] Notably, this surface polarization can also penetrate through the material and enhance the signal from bulk when appropriate conditions are met.[2]
The most common method for DNP-SENS implies impregnation of a solid material with a solution of tailored organic biradicals (e.g. TEKPol, AMUPol). At 100 K, under magic angle spinning (MAS) and constant microwave irradiation, polarization transfer occurs from the electron spins to surrounding nuclear spins (frozen glassy matrix) via cross-effect.[3] The surface of the solid is then hyperpolarized via spin diffusion through the solvent proton bath and a cross-polarization from the protons to another spin-1/2 nuclei (e.g. 13C, 15N etc.) or a quadrupolar nuclei, albeit much less efficiently.
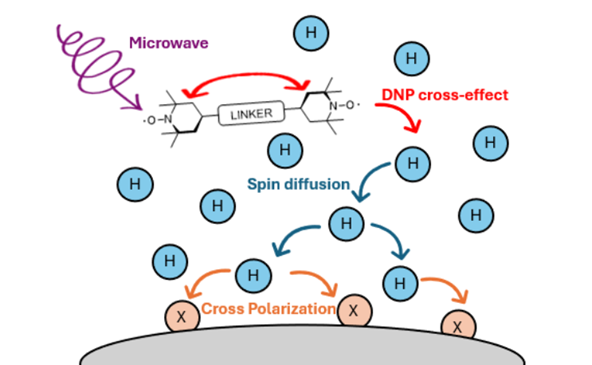
In the Copéret group, we conduct DNP-SENS measurement using 400 and 600 MHz spectrometers equipped with a 263 GHz klystron and 395 GHz gyrotron respectively, along with the necessary low temperature (LT) cabinets allowing to cool samples down to 100 K. Our instruments are equipped with 1.3 and 3.2 mm DNP probes, that are particularly designed to measure nuclei from 1H to very low gamma nuclei like 183W. In addition, we actively collaborate with research groups within ETH as well as with ENS Lyon (400, 800 MHz) and EPFL (900 MHz/Gyrotron) to explore novel DNP applications and methodologies[1] [4] [5] [6-8].

Earlier efforts within the “DNP-SENS consortium” (Copéret/Emsley/Lesage/Ouari/Tordo) have been devoted to improving protocols, comprising the development of tailored biradicals, characterization ranges of surface sites, in particular organic functionalities including ligands bound to metal sites, i.e. 13C and 15N, for large class of materials spanning from oxides (SiO2 [9, 10], Al2O3 [11, 12], silica-alumina [11]) to quantum dots [13] and more recently metal chlorides, e.g. MgCl2 supports for Ziegler-Natta catalysts.[5] A specific attention has been devoted to the development of protocols to avoid radical quenching by using either dendritic radicals or surface passivating agents like pyridine, that enabled characterization of olefin metathesis and polymerization catalysts[5, 8]. We also explore the development of new DNP-techniques. Most recently we have shown in collaboration with Emsley et al. that the 31P-NMR Signal of phosphine ligands in organometallic complexes can be enhanced (>100) at room temperature in solution by polarization transfer to the free ligand from an organic radical and subsequent ligand exchange.
The most recent studies have shown that DNP could also be applied to transition-metal sites:
1) Immobilized Pt sites on hybrid organic inorganic silica material with enhancements >90 for195Pt NMR. [14]
2) Grafted Ag-NHC sites on various oxide supports including silica, alumina, and silica-alumina via 105Ag NMR, a very challenging low γ-nuclei,[15] where DNP enhancements enabled determination of surface sites acidity since Ag+ and protons are isolobal.
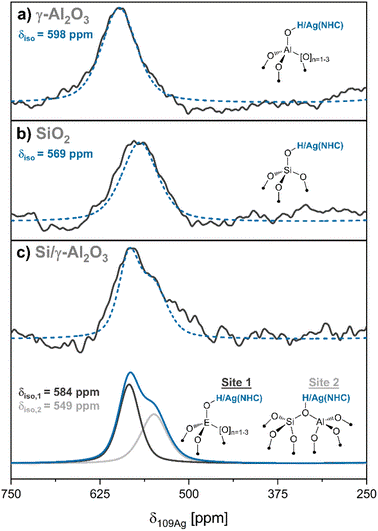
Selected Referneces
1. Rossini, A.J., et al., Dynamic Nuclear Polarization Surface Enhanced NMR Spectroscopy. Accounts of Chemical Research, 2013. 46(9): p. 1942-1951.
2. Björgvinsdóttir, S., et al., Bulk Nuclear Hyperpolarization of Inorganic Solids by Relay from the Surface. Journal of the American Chemical Society, 2018. 140(25): p. 7946-7951.
3. Maly, T., et al., Dynamic nuclear polarization at high magnetic fields. The Journal of Chemical Physics, 2008. 128(5): p. 052211.
4. Kubicki, D.J., et al., Rational design of dinitroxide biradicals for efficient cross-effect dynamic nuclear polarization. Chemical Science, 2016. 7(1): p. 550-558.
5. Yakimov, A.V., et al., A Formulation Protocol with Pyridine to Enable Dynamic Nuclear Polarization Surface-Enhanced NMR Spectroscopy on Reactive Surface Sites: Case Study with Olefin Polymerization and Metathesis Catalysts. The Journal of Physical Chemistry Letters, 2020. 11(9): p. 3401-3407.
6. Rao, Y., et al., Probing Homogeneous Catalysts and Precatalysts in Solution by Exchange-Mediated Overhauser Dynamic Nuclear Polarization NMR. Journal of the American Chemical Society, 2024. 146(18): p. 12587-12594.
7. Liao, W.-C., et al., Dynamic Nuclear Polarization Surface Enhanced NMR spectroscopy (DNP SENS): Principles, protocols, and practice. Current Opinion in Colloid & Interface Science, 2018. 33: p. 63-71.
8. Liao, W.-C., et al., Dendritic polarizing agents for DNP SENS. Chemical Science, 2017. 8(1): p. 416-422.
9. Kaeffer, N., D. Mance, and C. Copéret, N-Heterocyclic Carbene Coordination to Surface Copper Sites in Selective Semihydrogenation Catalysts from Solid-State NMR Spectroscopy. Angewandte Chemie International Edition, 2020. 59(45): p. 19999-20007.
10. Tensi, L., et al., Single-Site Iridium Picolinamide Catalyst Immobilized onto Silica for the Hydrogenation of CO2 and the Dehydrogenation of Formic Acid. Inorganic Chemistry, 2022. 61(27): p. 10575-10586.
11. Valla, M., et al., Atomic Description of the Interface between Silica and Alumina in Aluminosilicates through Dynamic Nuclear Polarization Surface-Enhanced NMR Spectroscopy and First-Principles Calculations. Journal of the American Chemical Society, 2015. 137(33): p. 10710-10719.
12. Moroz, I.B., et al., Discerning γ-Alumina Surface Sites with Nitrogen-15 Dynamic Nuclear Polarization Surface Enhanced NMR Spectroscopy of Adsorbed Pyridine. The Journal of Physical Chemistry C, 2018. 122(20): p. 10871-10882.
13. Piveteau, L., et al., Resolving the Core and the Surface of CdSe Quantum Dots and Nanoplatelets Using Dynamic Nuclear Polarization Enhanced PASS–PIETA NMR Spectroscopy. ACS Central Science, 2018. 4(9): p. 1113-1125.
14. Wang, Z., et al., Speciation and Structures in Pt Surface Sites Stabilized by N-Heterocyclic Carbene Ligands Revealed by Dynamic Nuclear Polarization Enhanced Indirectly Detected 195Pt NMR Spectroscopic Signatures and Fingerprint Analysis. Journal of the American Chemical Society, 2022. 144(47): p. 21530-21543.
15. Hansen, C., et al., 109Ag NMR chemical shift as a descriptor for Brønsted acidity from molecules to materials. Chemical Science, 2024. 15(8): p. 3028-3032.
16. Lätsch, L., E. Lam, and C. Copéret, Electronegativity and location of anionic ligands drive yttrium NMR for molecular, surface and solid-state structures. Chemical Science, 2020. 11(26): p. 6724-6735.