Dehydrogenation
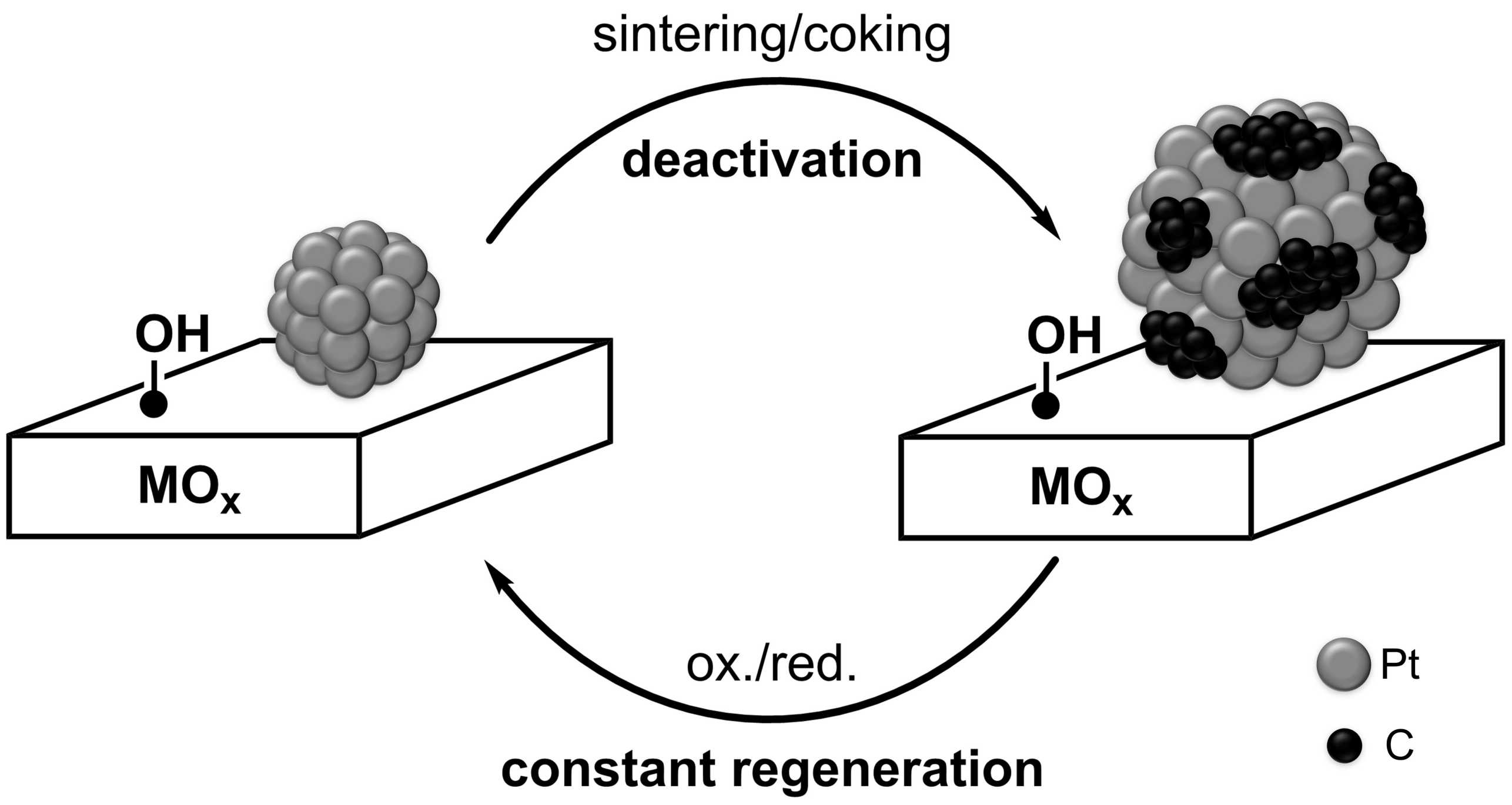
The large-scale exploitation of shale gas has led to switching the industrial process to produce light alkenes from naphtha-based cracking to the direct dehydrogenation of light alkanes (on-purpose olefin production). In addition, the increasing demand of light alkenes, which is used for the production of polymer and bulk chemicals, has pushed to increase the capacity in dehydrogenation process and to develop more efficient catalysts. Since alkane dehydrogenation is highly endothermic, this process requires high temperatures to proceed. For example, in case of propane dehydrogenation - PDH -, the operating temperature is usually above 550 °C to achieve reasonable conversion, 30-45% depending on exact conditions. Such harsh conditions result in undesired side reactions, e.g. thermal cracking, as well as fast catalyst deactivation due to coking and sintering (Figure 1). Therefore, periodic regeneration cycles, constituted of subsequent oxidation and reduction steps, are applied to remove coke and reactivate the catalysts.
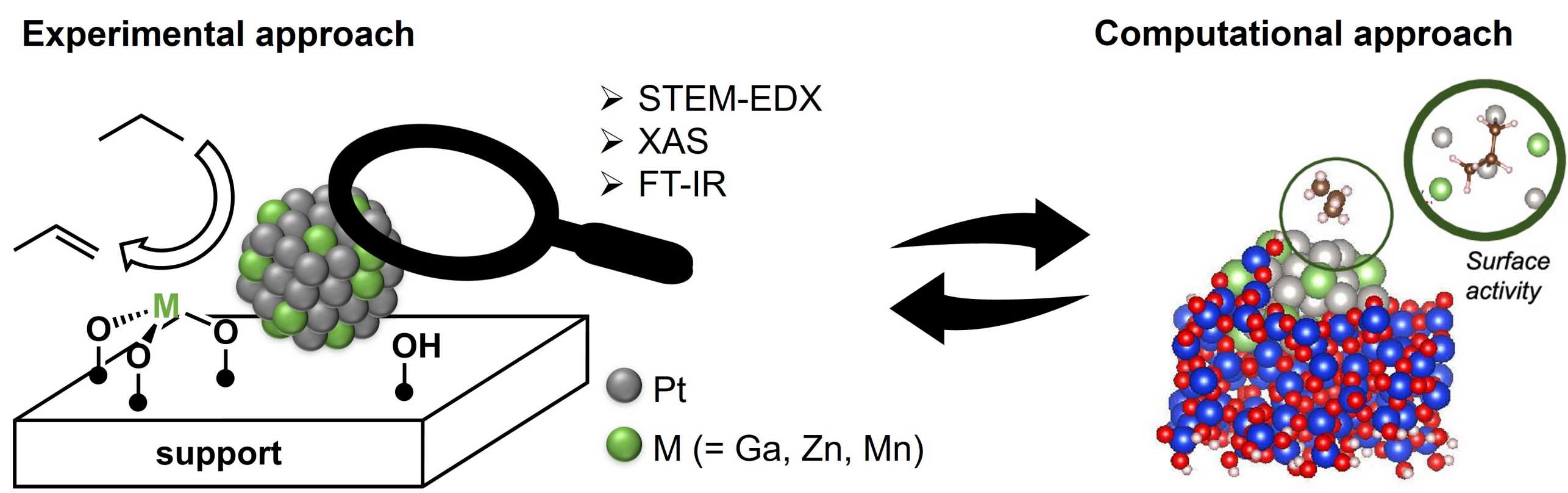
In industrial PDH processes, two main catalytic systems, CrxO/Al2O3 and Pt-Sn/Al2O3, have been used for the Lummus CATOFIN and UOP Oleflex processes, respectively. In Pt-based catalytic systems, a second metal dopant typically Sn is used in order to enhance catalyst productivity and stability. Alternative dopants or so-called promoters have emerged such as Ga, In, Zn and more recently Mn. Notably, the role of dopants remains debated and their promotional effects are not fully understood.
In that context, our group has applied surface organometallic chemistry (SOMC ) and developed a series of highly efficient PDH catalysts based on Pt-M bimetallic systems (M = Ga, Zn and Mn) on various supports (Figure 2). The thus-prepared catalysts are typically more aimable to detailed characterization; for instance, scanning transmission electron microscopy (STEM ) coupled with energy dispersive X-ray spectroscopy (EDX/EDS) indicates the formation of Pt-M alloyed nanoparticles with narrow distribution in all cases, while X-ray absorption spectroscopy (XAS ) and CO adsorption study by FT-IR indicate that these bimetallic catalysts are composed of alloyed nanoparticles. These detailed characterizations augmented by computational studies relying on Ab Initio Metadynamics have revealed that Pt site isolation in the secondary metal (Sn, Ga…) and strong metal support interaction contribute to the high selectivity (by avoiding coking) and greater stability (by avoiding sintering). Current directions in this area focus on understanding the role of promoters and supports on catalyst stability upon regeneration.
References:
Reviews:
[1] Docherty, S. R.; Rochlitz, L.; Payard, P.-A.; Copéret, C. Chem. Soc. Rev. 2021, 50, 5806-5822.
[2] Monai, M.; Gambino, M.; Wannakao, S.; Weckhuysen, B. M. Chem. Soc. Rev. 2021, 50, 11503-11529.
Recent primary publications from our group on this topic:
[3] Searles, K.; Chan, K. W.; Mendes Burak, J. A.; Zemlyanov, D.; Safonova, O.; Copéret, C. J. Am. Chem. Soc. 2018, 140, 11674-11679.
[4] Rochlitz, L.; Searles, K.; Alfke, J.; Zemlyanov, D.; Safonova, O. V.; Copéret, C.. Chem. Sci. 2020, 11, 1549-1555.
[5] Payard, P. A.; Rochlitz, L.; Searles, K.; Foppa, L.; Leuthold, B.; Safonova, O. V.; Comas-Vives, A.; Copéret, C. JACS Au 2021, 1, 1445-1458.
[6] Rochlitz, L.; Pessemesse, Q.; Fischer, J. W. A.; Klose, D.; Clark, A. H.; Plodinec, M.; Jeschke, G.; Payard, P.-A.; Copéret, C. J. Am. Chem. Soc. 2022, 144, 13384-13393.
[7] Rochlitz, L.; Fischer, J. W. A.; Pessemesse, Q.; Clark, A. H.; Ashuiev, A.; Klose, D.; Payard, P.-A.; Jeschke, G.; Copéret, C. JACS Au 2023, 3, 1939-1951.
[8] Brack, E.; Plodinec, M.; Willinger, M.-G.; Copéret, C. Chem. Sci. 2023, 44, 12739-12746.