Polymerization
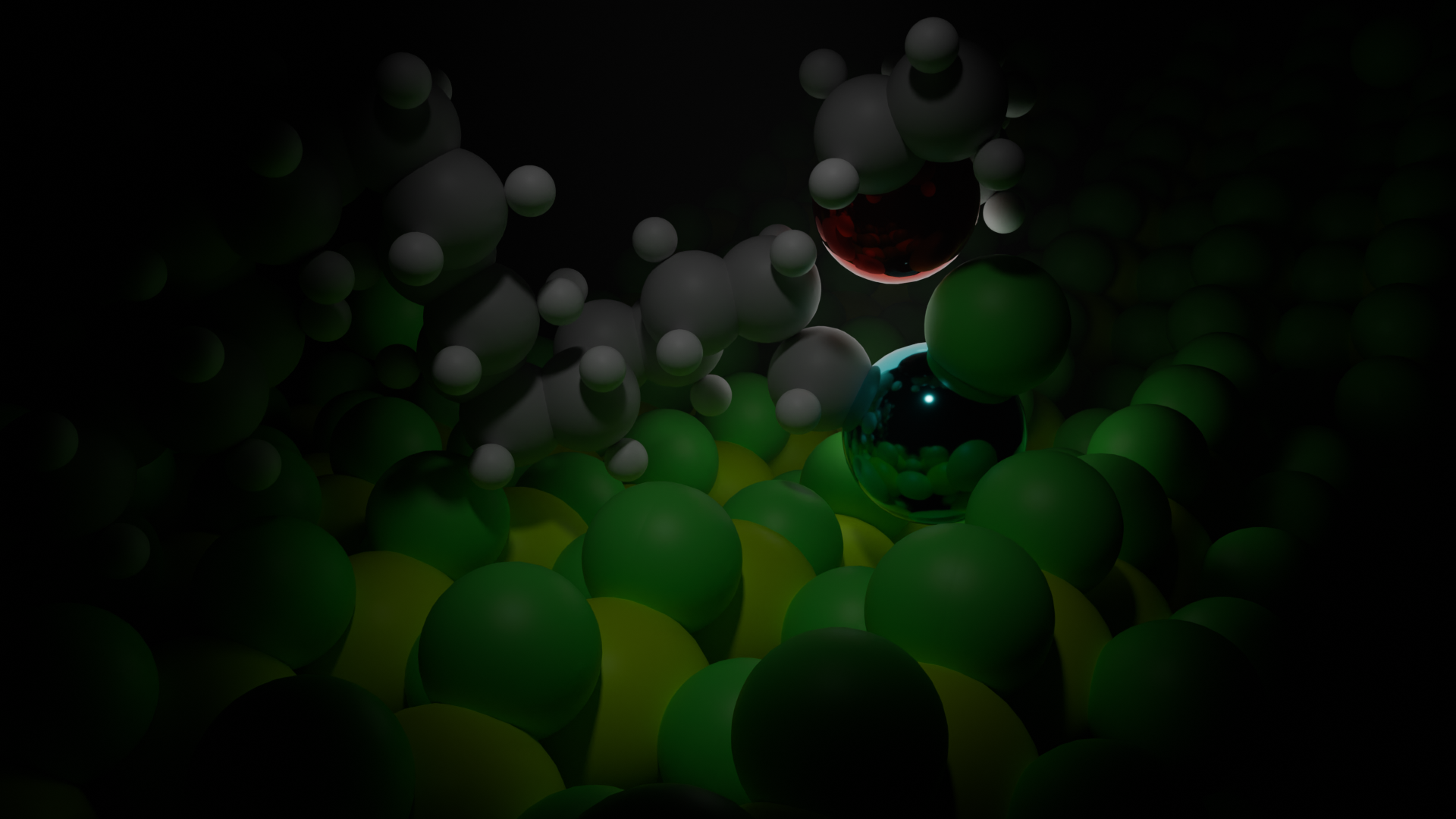
Toward Molecular-level Understanding of Industrial Catalysts
Polymers have become an essential part of modern society from food-packaging, medical supplies to construction materials including window frames and cars. Polyolefins are one of the most common family of polymers, [1] and their production largely relies on Ti-based Ziegler-Natta (TiCl4/MgCl2) catalysts, annually producing >50% of polyethylene (PE) and >90% of polypropylene (PP) in the world. Another large fraction of PE (>40%) is made with Cr-based Phillips catalysts (CrO3/SiO2). While developed since the fifties, the nature of the active sites and detailed mechanism of these catalysts remain elusive despite their tremendous importance in the polymer industry and in fine in our society. Following our work on single-site catalysts, our group has also studied these ill-defined and key industrial polymerization catalysts with the goal of bringing molecular-level understanding of these systems as well as the related Union Carbide catalysts (Cp2Cr@SiO2).
Towards this goal, we use surface organometallic chemistry (SOMC)2 to develop molecularly-defined surface analogs of these industrial catalysts. Combined with state-of-the-art spectroscopic techniques augmented by computational studies, we address the nature of the activation sites and the mode of activation of industrial catalysts.
Modelling Catalyst Structures: from molecular analogues to Surface Organometallic Chemistry
To understand these complex systems, we take a molecular approach to mimic the structure of potential active intermediates and use spectroscopic tools to understand the electronic structure of these metal centers.
In context of Ziegler-Natta catalysts, we prepared for instance molecular and surface-bound Ti(III) alkyl species [3,4] that show high activity in olefin polymerization demonstrating that besides group 4 cationic species, Ti(III) alkyl species also highly competent to initiate and propagate polymerization. Detailed EPR spectroscopy complemented by DFT calculations, highlighted the importance of this specific electronic structure, favoring olefin insertion through the pi-character of the metal–alkyl bond and the spin-delocalization possible for d1 species. This finding parallels what we had observed for cationic metallocene polymerization catalysts, where pi-character of the Metal-Alkyl bond is key to efficient olefin insertion showing the formally olefin insertion is similar to a [2+2]-cycloaddition [5]. In parallel, the importance of radical character in the electronic structure of olefin polymerization catalysts was further highlighted by a lanthanoid-based highly active polymerization catalyst, Cp2Sm@SiO2. [6]
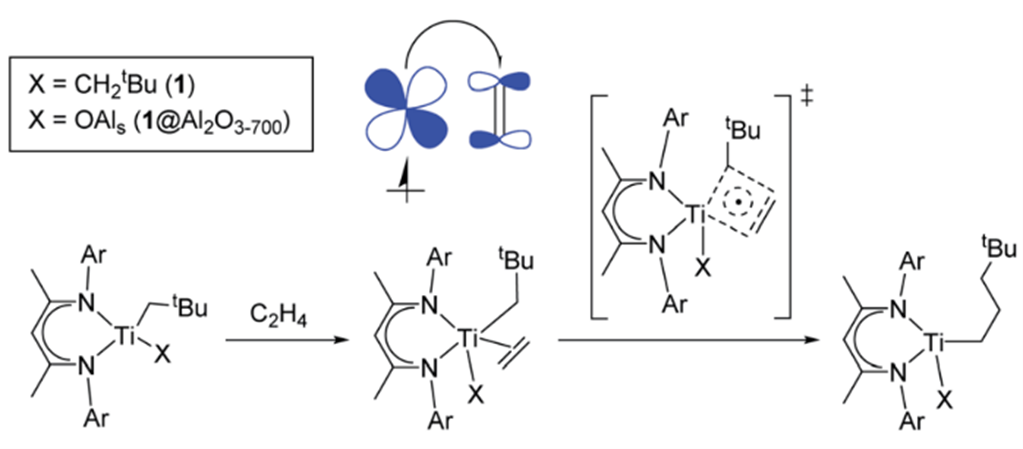
Regarding the Chromium-based polymerization catalysts, Phillips (CrO3@SiO2) and Union carbide (Cp2Cr@SiO2) catalysts, there has been animated debates regarding the nature of its active sites. [7] To address this, we developed a series of molecularly defined surface analogues of their putative active species – Cr(II/III) site – with tailored ligand environment highlighting the general excellent polymerization activity of Cr(III) alkyl species. [8–11]
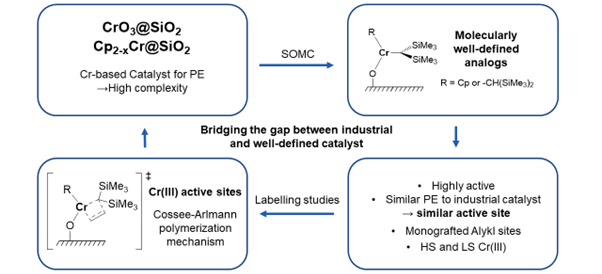
Spectroscopic Characterization of Industrial Catalysts
With the molecular insights from model systems in hand, advanced spectroscopic techniques have enabled us to tackle the nature of industry-relevant catalysts.
The Phillips catalyst features an entangled mixture of surface sites, that has been often tracked with Cr K-edge X-ray absorption spectroscopy (XAS), leading to many debates regarding spectral interpretation. Recent works based on large spectral libraries of Cr-compounds augmented with machine learning approaches have enabled us to advance spectral interpretation, [12] and to show that it is in fact difficult to distinguish Cr(II) and Cr(III) surface species without complementary data. Yet, detailed studies with molecular surface species prepared by SOMC enabled us to show that Cr(III) species form the most competent active sites, while Cr(II) needs to be oxidized to Cr(III) to become active. [8,9] Such a scenario was also found in the Union Carbide system, in which combined IR, EPR and paramagnetic NMR spectroscopy showcased the relevance of Cr(III) alkyl species. [10,13]
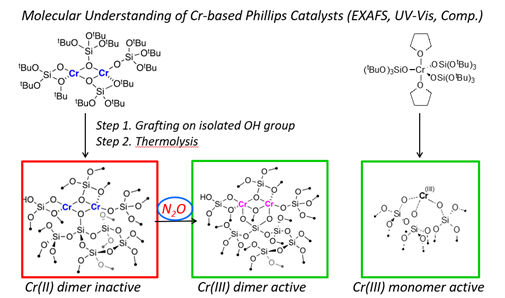
Regarding Ziegler-Natta catalysts, our group has focused on metal-centered spectroscopy, focusing on solid-state NMR and EPR spectroscopies to characterization the pre-catalysts and the activated catalysts respectively. Using metal-based 51V and 47/49Ti NMR, we have shown that the classical ZN pre-catalyst (TiCl4/MgCl2/THF) and its vanadium analogue (VOCl3/MgCl2/THF) actually contains well-defined metal sites. For the most studied Ti-based ZN catalysts, it was shown that the dispersion of TiCl4 on MgCl2 post-treated with BCl3 generate well-defined hexacoordinated sites surrounded by six chlorido ligands and likely adsorbed on an amorphous MgCl2 support. [14,15]
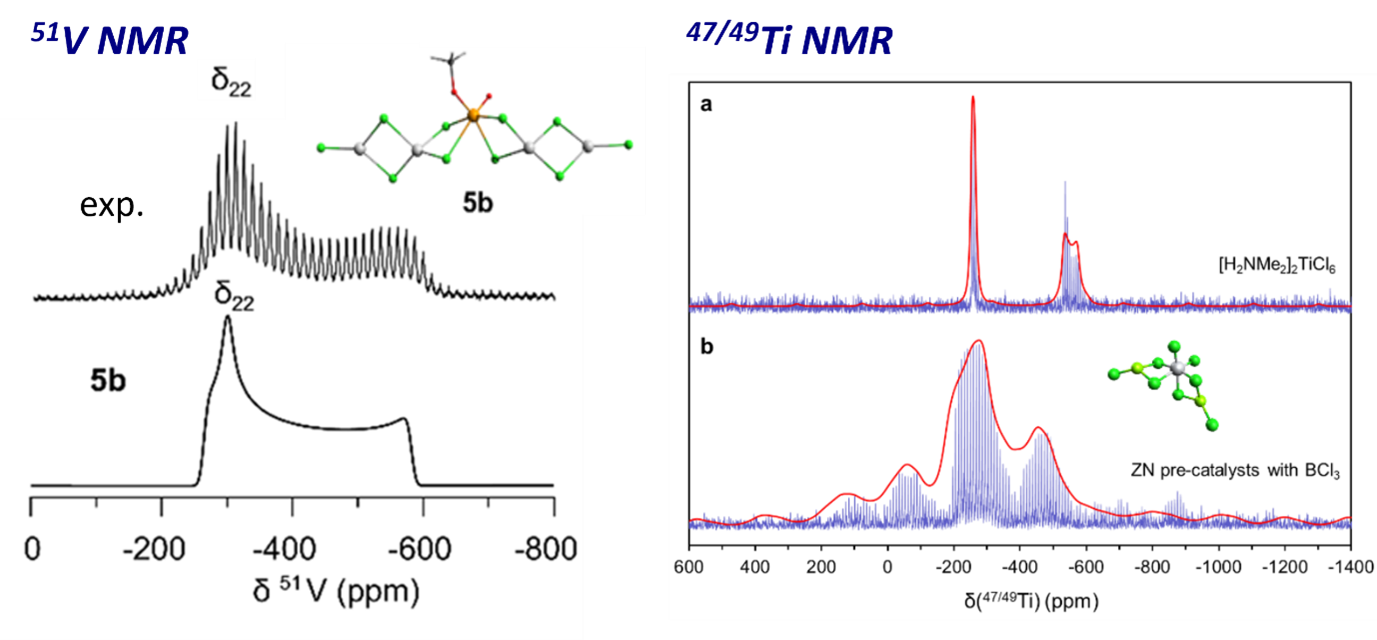
Upon activation with AlEt3, part of the sites are converted into the active Ti(III)–Alkylaluminium bimetallic sites along with inactive supported TiCl3. [16] As discussed with the molecular model above, these sites are ideally suited to polymerize the olefin via an augmented Cossee–Arlman mechanism highlighting the importance of the d1 electronic structure as discussed above.
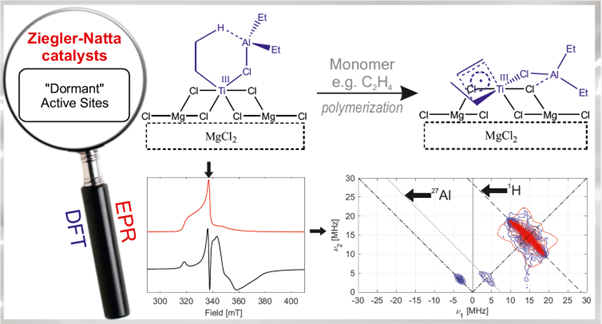
Chemical Recycling of Polyolefins
Polyolefins also represent nearly two-thirds of all post-consumer plastic waste. Therefore, we are also currently applying our expertise to develop chemical recycling methods to break down polymer waste.
Selected References
(1) Hoff, R. Handbook of Transition Metal Polymerization Catalysts; John Wiley & Sons, 2018.
(2) Copéret, C.; Comas-Vives, A.; Conley, M. P.; Estes, D. P.; Fedorov, A.; Mougel, V.; Nagae, H.; Núnez-Zarur, F.; Zhizhko, P. A. Surface Organometallic and Coordination Chemistry toward Single-Site Heterogeneous Catalysts: Strategies, Methods, Structures, and Activities. Chem. Rev. 2016, 116 (2), 323–421.
(3) Allouche, F.; Klose, D.; Gordon, C. P.; Ashuiev, A.; Wörle, M.; Kalendra, V.; Mougel, V.; Copéret, C.; Jeschke, G. Low-Coordinated Titanium(III) Alkyl-Molecular and Surface-Complexes: Detailed Structure from Advanced EPR Spectroscopy. Angew. Chem. Int. Ed Engl. 2018, 57 (44), 14533–14537.
(4) Ashuiev, A.; Allouche, F.; Wili, N.; Searles, K.; Klose, D.; Copéret, C.; Jeschke, G. Molecular and Supported Ti (III)-Alkyls: Efficient Ethylene Polymerization Driven by the π-Character of Metal--Carbon Bonds and Back Donation from a Singly Occupied Molecular Orbital. Chem. Sci. 2021, 12 (2), 780–792.
(5) Gordon, C. P.; Shirase, S.; Yamamoto, K.; Andersen, R. A.; Eisenstein, O.; Copéret, C. NMR Chemical Shift Analysis Decodes Olefin Oligo- and Polymerization Activity of D0 Group 4 Metal Complexes. Proc. Natl. Acad. Sci. U. S. A. 2018, 115 (26), E5867–E5876.
(6) Allouche, F.; Chan, K. W.; Fedorov, A.; Andersen, R. A.; Copéret, C. Silica-Supported Pentamethylcyclopentadienyl Ytterbium(II) and Samarium(II) Sites: Ultrahigh Molecular Weight Polyethylene without Co-Catalyst. Angew. Chem. Int. Ed Engl. 2018, 57 (13), 3431–3434.
(7) Groppo, E.; Martino, G. A.; Piovano, A.; Barzan, C. The Active Sites in the Phillips Catalysts: Origins of a Lively Debate and a Vision for the Future. ACS Catal. 2018, 8 (11), 10846–10863.
(8) Delley, M. F.; Conley, M. P.; Copéret, C. Polymerization on CO-Reduced Phillips Catalyst Initiates through the C-H Bond Activation of Ethylene on Cr-O Sites. Catal. Letters 2014, 144 (5), 805–808.
(9) Delley, M. F.; Núñez-Zarur, F.; Conley, M. P.; Comas-Vives, A.; Siddiqi, G.; Norsic, S.; Monteil, V.; Safonova, O. V.; Copéret, C. Proton Transfers Are Key Elementary Steps in Ethylene Polymerization on Isolated Chromium(III) Silicates. Proc. Natl. Acad. Sci. U. S. A. 2014, 111 (32), 11624–11629.
(10) Trummer, D.; Nobile, A. G.; Payard, P.-A.; Ashuiev, A.; Kakiuchi, Y.; Klose, D.; Jeschke, G.; Copéret, C. Union Carbide Polymerization Catalysts: From Uncovering Active Site Structures to Designing Molecularly-Defined Analogs. Chem. Sci. 2022, 13 (37), 11091–11098.
(11) Ashuiev, A.; Giorgia Nobile, A.; Trummer, D.; Klose, D.; Guda, S.; Safonova, O. V.; Copéret, C.; Guda, A.; Jeschke, G. Active Sites in Cr(III)‐based Ethylene Polymerization Catalysts from Machine‐learning‐supported XAS and EPR Spectroscopy. Angew. Chem. Weinheim Bergstr. Ger. 2024, 136 (1). https://doi.org/10.1002/ange.202313348.
(12) Trummer, D.; Searles, K.; Algasov, A.; Guda, S. A.; Soldatov, A. V.; Ramanantoanina, H.; Safonova, O. V.; Guda, A. A.; Copéret, C. Deciphering the Phillips Catalyst by Orbital Analysis and Supervised Machine Learning from Cr Pre-Edge XANES of Molecular Libraries. J. Am. Chem. Soc. 2021, 143 (19), 7326–7341.
(13) Nobile, A. G.; Trummer, D.; Berkson, Z. J.; Wörle, M.; Copéret, C.; Payard, P.-A. Assigning 1H Chemical Shifts in Paramagnetic Mono- and Bimetallic Surface Sites Using DFT: A Case Study on the Union Carbide Polymerization Catalyst. Chem. Sci. 2023, 14 (9), 2361–2368.
(14) Yakimov, A. V.; Kaul, C. J.; Kakiuchi, Y. Well-Defined Ti Surface Sites in Ziegler–Natta Pre-Catalysts from 47/49Ti Solid-State NuclearMagnetic Resonance Spectroscopy. The Journal of 2024.
(15) Sabisch, S.; Kakiuchi, Y.; Docherty, S. R. Geometry and Local Environment of Surface Sites in Vanadium-Based Ziegler–Natta Catalysts from 51V Solid-State NMR Spectroscopy. Journal of the 2023.
(16) Ashuiev, A.; Humbert, M.; Norsic, S.; Blahut, J.; Gajan, D.; Searles, K.; Klose, D.; Lesage, A.; Pintacuda, G.; Raynaud, J.; Monteil, V.; Copéret, C.; Jeschke, G. Spectroscopic Signature and Structure of the Active Sites in Ziegler-Natta Polymerization Catalysts Revealed by Electron Paramagnetic Resonance. J. Am. Chem. Soc. 2021, 143 (26), 9791–9797.